ORP: The Highly Misinterpreted Concept and Its Implications on In Situ Remediation of Contaminants in the Subsurface
The influence of ORP on the bioremediation of contaminants in the subsurface
Environmental remediation, especially in situ bioremediation, represents a pivotal strategy for the sustainable management of contaminated sites. A critical, yet often misinterpreted, factor in optimizing these efforts is the role of Oxidation-Reduction Potential (ORP) in influencing microbial processes. This article aims to demystify ORP and explore its implications for the in situ remediation of various contaminants, including chlorinated compounds, petroleum hydrocarbons, heavy metals, and manganese.
Understanding ORP and Microbial Activity
ORP, measured in millivolts (mV), indicates a system’s tendency towards oxidation or reduction reactions, profoundly impacting microbial community structure and activity. Highly reducing conditions (-400 mV to -200 mV) favor anaerobic processes led by methanogens and sulfate-reducing bacteria, while oxidizing conditions (200 mV to 400 mV) promote aerobic bacteria and chemolithoautotrophs’ activities (Jones and Hallin, 2010). Figure 1 below illustrates the various microbially mediated processes across the ORP range in the environment.
Implications for In-Situ Remediation
Chlorinated Compounds
For chlorinated solvents like PCE and TCE, methanogenesis under highly reducing conditions (ORP: -300 mV to -200 mV) is crucial for reductive dechlorination. Methanogens such as Methanobacterium and Methanosarcina species, alongside dechlorinating bacteria like Dehalococcoides and Dehalobacter species, play pivotal roles in this process (Smith et al., 2015). Managing the delicate balance between methanogenesis and dechlorination, substrate competition, and ensuring anaerobic conditions are essential for effective remediation.
Petroleum Hydrocarbons
Petroleum hydrocarbon remediation often requires addressing anaerobic biodegradation pathways, especially in subsurface environments where oxygen is limited. The ORP plays a critical role in determining the feasibility and efficiency of these pathways:
- Iron Reduction (ORP: -150 mV to 0 mV): Ferric iron [Fe(III)] can serve as an electron acceptor for the anaerobic oxidation of hydrocarbons. Geobacter and Shewanella species are known to facilitate this process (Lovley et al., 1996).
- Manganese Reduction (ORP: -100 mV to 0 mV): Manganese-reducing bacteria can facilitate the reduction of manganese compounds, transforming them into less soluble and less toxic forms.
- Nitrate Reduction (ORP: +200 mV to +350 mV): Nitrate-reducing bacteria such as Pseudomonas and Paracoccus species can mediate the anaerobic degradation of hydrocarbons, using nitrate as the electron acceptor (Rabus et al., 2006).
- Oxygen Reduction (Aerobic Respiration): Under aerobic conditions, oxygen serves as the final terminal electron acceptor, converting hydrocarbons to water and carbon dioxide. This process is facilitated by aerobic bacteria such as Pseudomonas and Acinetobacter species.
These processes, occurring at different ORP levels, showcase the adaptability of microbial communities to degrade hydrocarbons even in the absence of oxygen. Figure 2 shows the typical scenario of redox zone zones and biochemical processes in a hydrocarbon plume moving through an aquifer. A plume moving with groundwater flow typically will develop distinct redox zones as electron acceptors get depleted from the highest energy to the lowest energy (EPA 2000).
Heavy Metals
The remediation of heavy metals through bioremediation involves either mobilization or immobilization, significantly influenced by ORP:
- Reducing Conditions (ORP: -200 mV to 0 mV): Under these conditions, metal-reducing bacteria such as Geobacter and Shewanella species can facilitate the transformation of metals like Chromium [Cr(VI)] to less toxic forms [Cr(III)].
- Oxidizing Conditions (ORP: +200 mV to +400 mV): Conditions may facilitate the conversion of metals into more mobile or less toxic states, though specific microbial taxa involved would vary based on the metal and environmental conditions.
Navigating Misinterpretations
The complexity of ORP’s influence on microbial degradation processes underscores the necessity of accurate interpretation and application in remediation strategies. Misinterpretations of ORP data can lead to ineffective remediation efforts, underscoring the need for comprehensive monitoring and a deep understanding of subsurface microbial ecology.
Recommendations for Practitioners
- Rigorous ORP Monitoring: Continuous, precise monitoring of ORP values is critical for guiding the adjustment of bioremediation strategies to favor desired microbial processes.
- In-depth Microbial Community Analysis: Advanced molecular techniques should be employed to characterize microbial communities, focusing on identifying functional groups capable of degrading specific contaminants under varying ORP conditions.
- Strategic Substrate Management: The careful selection and management of substrates to support targeted microbial processes are essential, considering the ORP conditions and the specific contaminants of concern.
Conclusion
ORP’s role in microbial degradation processes is a critical yet often misunderstood aspect of in situ bioremediation. By demystifying ORP and leveraging insights into its implications for contaminant remediation, environmental professionals can enhance the efficiency and effectiveness of efforts to restore contaminated sites.
References
• Jones, C., & Hallin, S. (2010). Ecological and evolutionary factors underlying global and local assembly of denitrifier communities. Ecology Letters, 13(6), 667-674.
• Lovley, D. R., Coates, J. D., Blunt-Harris, E. L., Phillips, E. J. P., & Woodward, J. C. (1996). Humic substances as electron acceptors for microbial respiration. Nature, 382(6590), 445-448.
• Rabus, R., Boll, M., Heider, J., Meckenstock, R. U., Buckel, W., Einsle, O., … & Widdel, F. (2006). Anaerobic microbial degradation of hydrocarbons: From enzymatic reactions to the environment. Journal of Molecular Microbiology and Biotechnology, 11(5), 212-226.
• Widdel, F., & Rabus, R. (2001). Anaerobic biodegradation of saturated and aromatic hydrocarbons. Current Opinion in Biotechnology, 12(3), 259-276.
• Zengler, K., Richnow, H. H., Rosselló-Mora, R., Michaelis, W., & Widdel, F. (1999). Methane formation from long-chain alkanes by anaerobic microorganisms. Nature, 401(6750), 266-269.
This article seeks to clarify the critical role of ORP in microbial degradation processes relevant to in situ bioremediation, aiming to enhance the understanding and application of ORP in environmental remediation strategies. For further discussion or consultation on environmental remediation projects, feel free to connect.
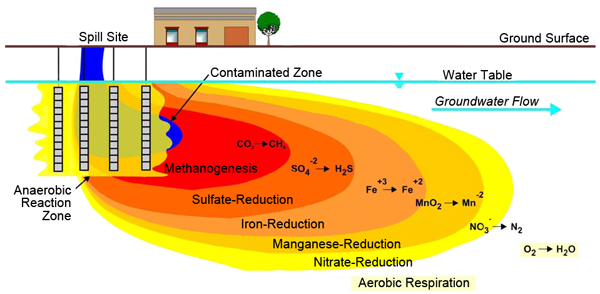
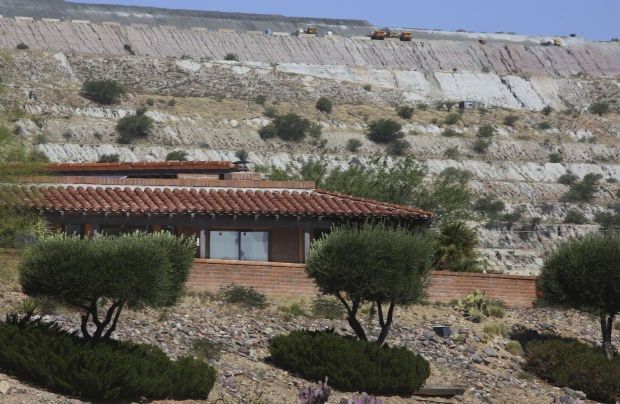
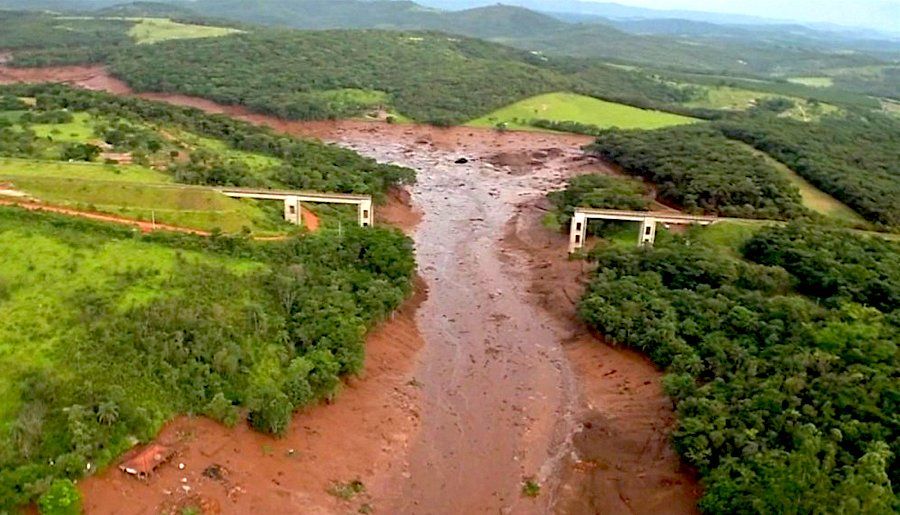
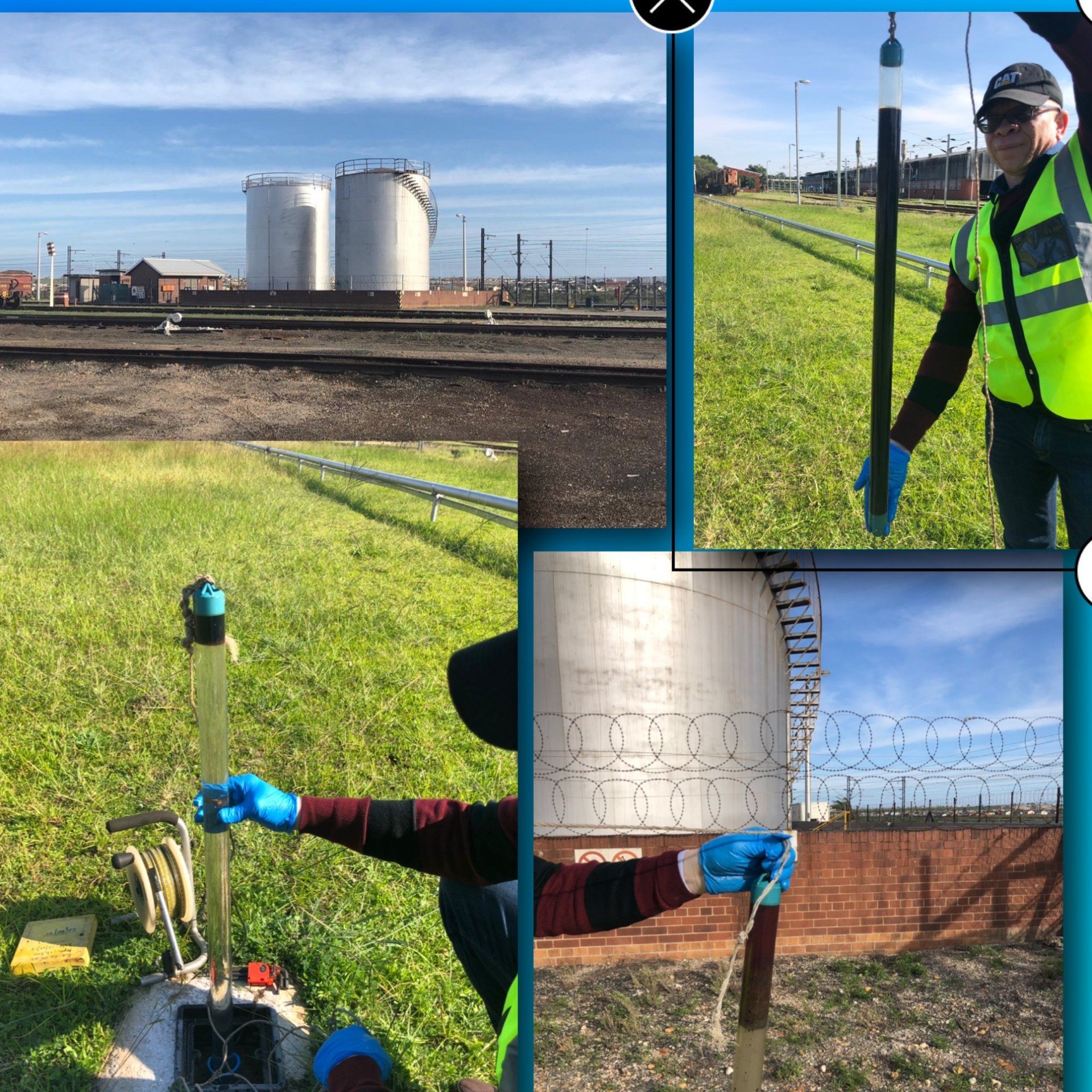
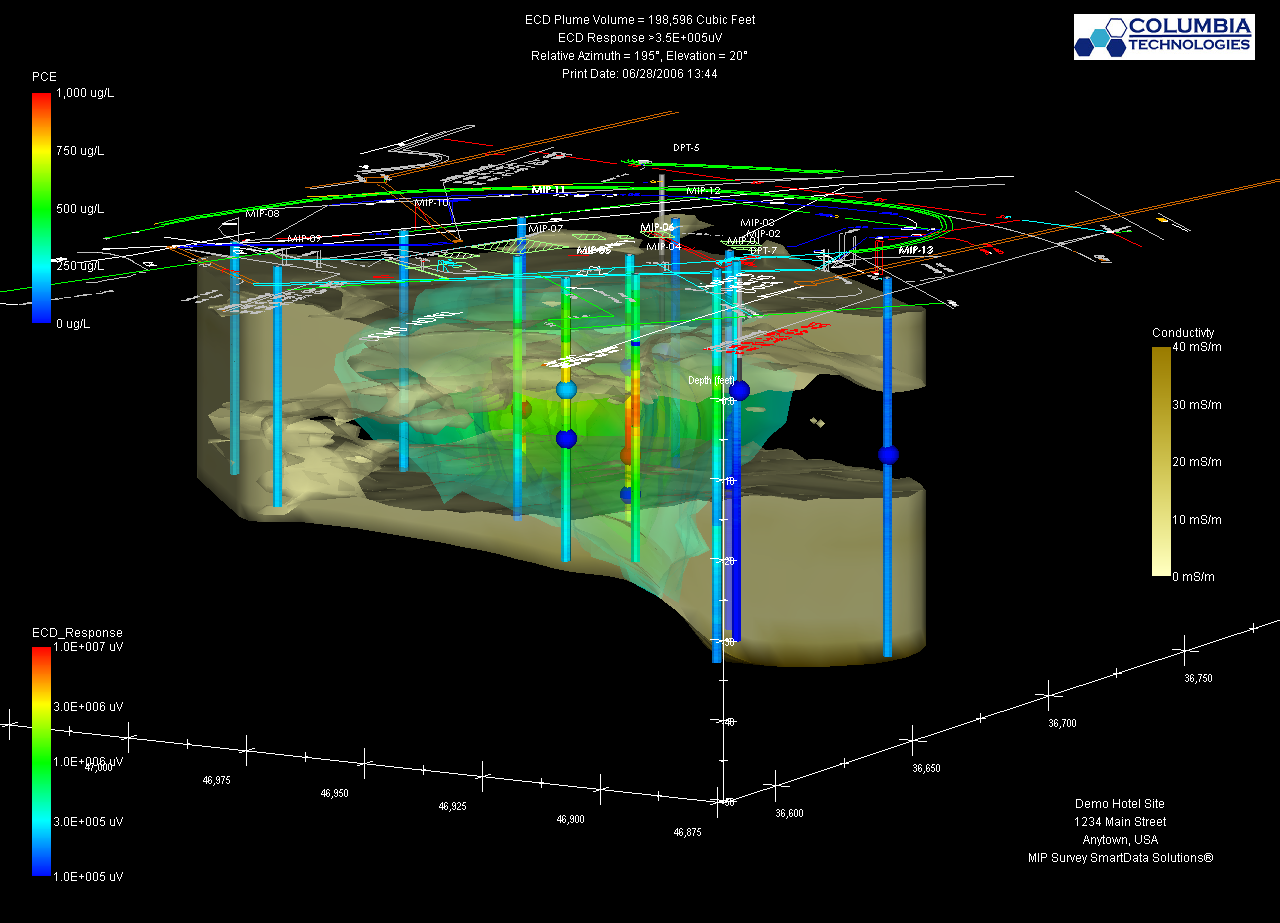
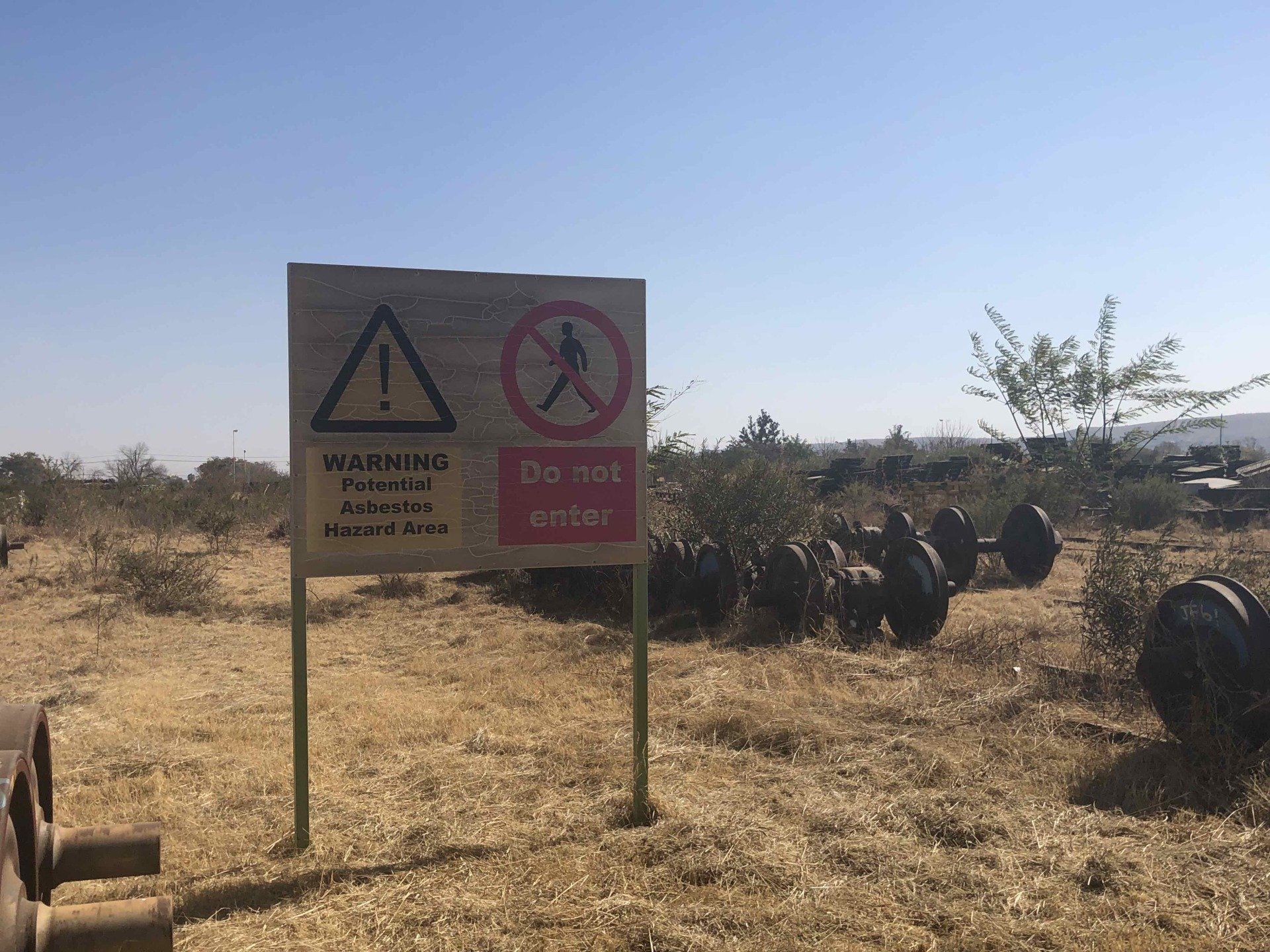
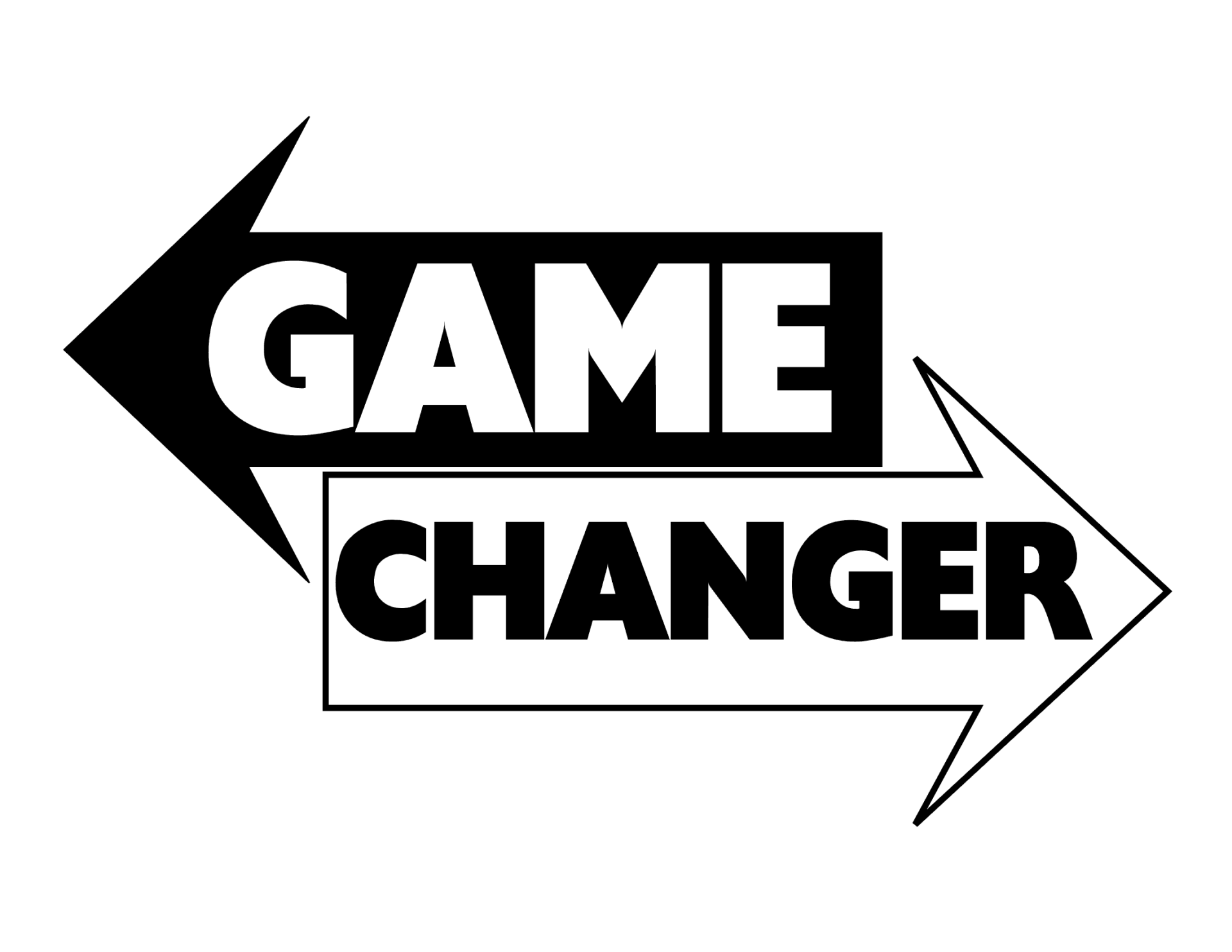
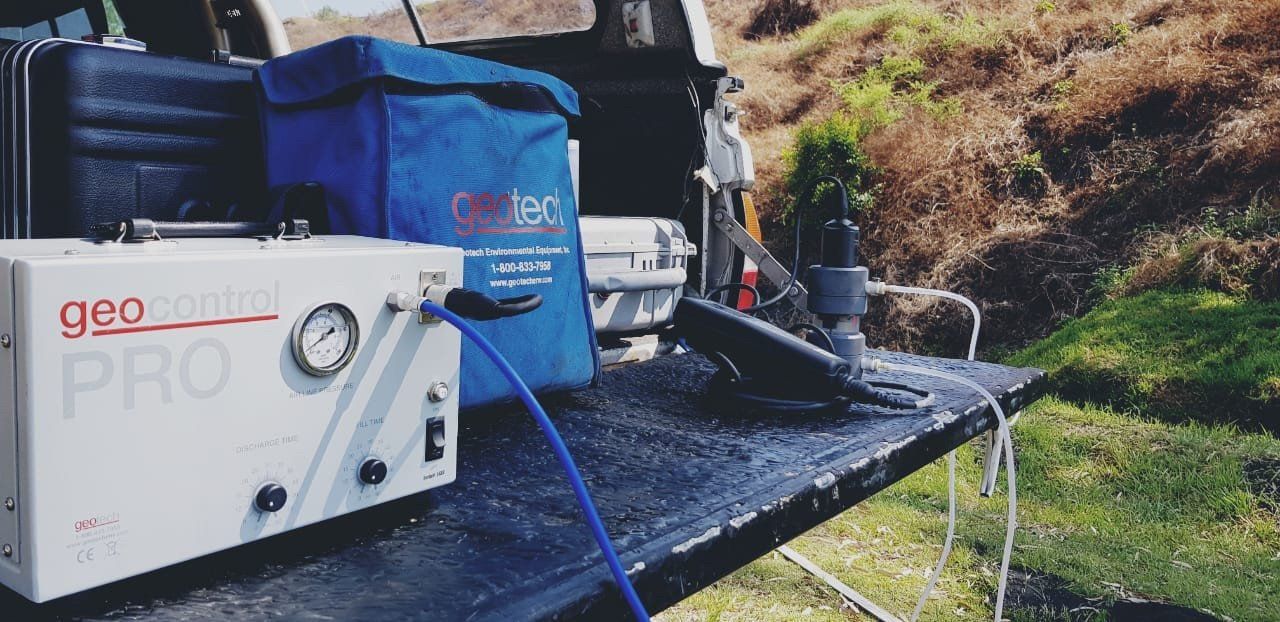